ARTICLES (6)
Advances in Nuclear Monitoring Technologies
Brent K. Park, John M. Blackadar, Ian H. Campbell, James D. Danneskiold,
Robert J. Estep, Gary Hal Gardner, M. William Johnson, Manuel D. Martinez,
Edward A. McKigney, William L. Myers, Clair J. Sullivan, Gregory J. Van Tuyle
Abstract: This article is based on an invited presentation by Brent Park of Los Alamos National Laboratory on March 13, 2006, at the American Physical Society meeting in Baltimore. The presentation focused on recent efforts in new detector materials and detector systems, as well as improving existing systems for homeland security.
I. Introduction
The problem of detecting an attempt to smuggle a nuclear weapon into the United States has not been solved and the detonation of such a device would be a catastrophe that would dwarf the terrible tragedy of September 11, 2001. It is estimated that annually ~7 million cargo containers enter the United States by sea and ~9 million by land.1 Twice in recent years, 7-kg chunks of depleted uranium (DU) — harmless itself but massive enough to resemble threatening quantities of weapons-grade uranium — are known to have passed border inspection without detection.2, 3 Clearly we need to substantially improve nuclear monitoring technologies, while acknowledging the extreme challenges of monitoring nuclear and radiological materials and differentiating the bad (weapons-useable) from the good (medical, industrial). In March 2006, major news outlets carried such headlines as “Dirty bomb test exposes security lapses: False radiation alarms are common – sometimes occurring more than 100 times a day,” describing how the Government Accounting Office was able to smuggle radioactive sources through multiple ports of entry into the United States without detection.4 The gamma radiation emitted by a threat is not readily distinguished from that emitted by many innocent items of commerce, and, as in this example, such signals are frequently ignored. Thus, reducing the false alarm rate is essential for a robust detection system.
II. Discussion
Protecting the United States against terrorists bringing in weapons-useable nuclear and radioactive materials requires advanced, robust detector systems that provide maximum detection efficiency with low false alarm rates, in addition to accurate radiation identification capabilities. Additional constraints include the need for detectors that are inexpensive, wide-area ranging, field ruggedized, and capable of near real-time data acquisition and analysis. In this paper, we provide a brief overview of recent efforts on nano-composite scintillators, quantum dots as detector materials, a microcalorimeter array for ultra-high-resolution spectrometry, and ongoing improvements to CdZnTe and high-pressure Xenon detectors. We comment on the limitations of Compton imagers for passive detection of nuclear signatures. We also examine active interrogation techniques and algorithm development work aimed at reducing false-alarm rates.
II-A. Advanced detector materials
To make passive gamma-ray detectors ubiquitous and useful, it is necessary to substantially reduce their cost while increasing detector volume and energy resolution. Improved energy resolution enables reliable isotope identification, thus separating threats from non-threats in a variety of situations. Many types of gamma and neutron-detector materials are available: Ge, CdZnTe, NaI, Plastic, and other scintillator materials. In the following table we summarize different detector material performances and costs:
Detector Material | Energy Resolution (% @ 662 keV) | Density (g/cc) | Estimated Cost ($/cc) | Maximum Volume (cc) |
Ge | 0.13 | 5.3 | 150 | 750 |
CdZnTe | 1.7 | 6.0 | 4400 | 3.4 |
NaI | 6 | 3.7 | 2 | 4200 |
Plastic Scintillator | Very Poor | 1.0 | 0.16 | 14000 |
Table 1. Comparison of commonly used detector materials. Numbers are approximate since performance/cost depend on specific detector design.
A key goal is to combine the performance characteristics shown in bold face with in a single material. One way to achieve this is to create a composite scintillator, where the materials are synthesized by industrial chemical means, with processing similar to plastic scintillators. Nano-composite material is one such example. Nano-sized particles of known insulating and semiconducting scintillators may be used as components of composite materials. Nano-composites can be used for passive detection and active interrogation and may offer improved energy resolution, due to the fact that nano-composites have reduced self-absorption and enhanced light-yield from reduced dimensionality, along with fast lifetime. Because nano-materials are synthesized in a scalable way, significant cost savings compared to bulk materials should be possible. Recently LANL developed techniques to produce nano-particle material.5 Several transparent pieces of a few-millimeters diameter were successfully manufactured, optically characterized and tested. Transparent samples have been produced with dimensions up to about 1 mm. We plan to scale up the production process to larger sizes of more practical interest and are evaluating the possibility of fabricating high-quantum-efficiency, large-area, low-cost organic photodiodes.
Another effort seeks to develop a new class of radiation-detection materials — composite inorganic semiconductor quantum dot/organic semiconductors — that possess the cost and processing advantages of organic scintillators and the ionization characteristics of inorganic semiconductors. Organic scintillators are widely used in radiation-detection applications due to their low cost, ease of fabrication, and fast response times. However, because their large and nonlinear ionization energy make them unsuitable for applications that require high-energy resolution or detection of strongly ionizing particles. The ionization energy of a semiconductor quantum dot is expected to be less than the bulk material and may approach the energy gap of the quantum dot.6
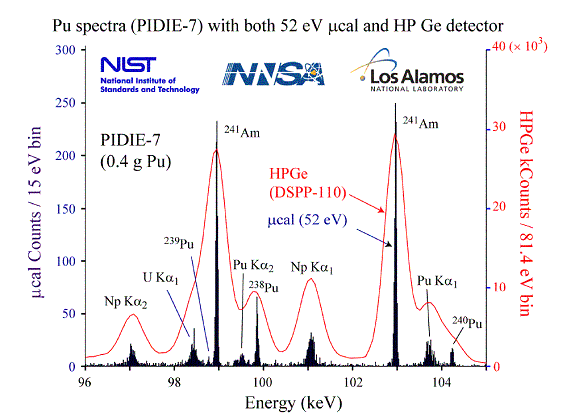
Figure 1. Microcalorimeter (black) and high-purity germanium (red) spectra of a mixture of plutonium isotopes.7 Minimal thermal noise is achieved at 100 mK. High sensitivity is due to use of a superconducting quantum interference device.
Microcalorimeters and bolometers made from thin superconducting films cooled to temperatures near 100mK provide unprecedented energy resolution (see Figure 1). A research team from LANL and the National Institute of Standards and Technology (NIST) has accurately measured the x- and gamma-ray spectra of plutonium samples with a pin-size calorimeter, achieving an energy resolution of 42 eV FWHM at 103 keV, roughly 10 times better than high-purity germanium detectors.7 Such measurements are valuable for non-destructive assays of radioactive isotopes mixed in with other materials. Successful development and implementation of the detectors is directly relevant to problems in nuclear forensics, international and domestic safeguards, attribution, and analysis of environmental samples. LANL and NIST plan to develop a microcalorimeter-based gamma-ray spectrometer with a count rate > 2- kHz and a sensitive area of 10 cm2. Such an instrument would provide new capabilities for analyzing such nuclear materials as mixed plutonium isotopes. Over the next two years, the collaboration seeks to make the detector system portable and ruggedized for field use.
II-B. Efforts to improve existing detectors and materials
CdZnTe is a semiconductor material used to detect various energy levels of radiation. CdZnTe detectors produce high-quality data in portable systems that are not sensitive to extreme variations in temperature or environment.8 Using such a detector, operators can identify whether a radiation source is near, its proximity, its constituent radioactive materials and their quantities, based on data collected by the detector and analyzed by an on-board microcomputer.8 One challenge to wider use of CdZnTe is its limited size and volume of a few cm. New configurations of CdZnTe detectors recently have been evaluated for nuclear material monitoring applications. A LANL team used two CdZnTe detectors, mounted side by side in a single housing behind slit collimators, and achieved about 3.5% energy resolution in unattended monitoring of UF6 enrichment.9 For this application, measurements of gamma-ray lines at 143 and 186 keV are used to deduce gas pressure. Due to their relatively high sensitivity coupled with low-power requirements, it may be possible to use CdZnTe detectors for remote monitoring of nuclear signatures for extended periods.
High-Pressure Xenon (HPXe) Detector: A recent demonstration at LANL showed HPXe detector performance may be superior to that of NaI, plastic scintillators, high-purity germanium, or lanthanum halide/bromide detector materials for many homeland security applications. A prototype HPXe detector showed good temperature stability. Its statistical energy resolution limit is better than 1% and it can provide real-time isotope identification capabilities and push the low-level detection limits.10 There are challenges in the handling and transporting of pressurized Xe gas. Such a detector shows a huge potential for accommodating unusual shapes for special applications as well as scalability to large sizes, but it is not yet cost-effective for wide use.
Compton Gamma-Ray Imager/Camera: Development of a passive gamma-ray imager includes efforts to use the Compton effect to diagnose the internal details of a suspicious device by passive detection alone. Although Compton cameras have been successfully used in astrophysics, using Compton cameras to detect passive nuclear signatures is a difficult challenge due to the inherent inefficiency of a device that requires double scattering. Most Compton imagers use several-hundred-micron-thick layers of pixilated silicon detectors followed by an array of CsI scintillators. The probability of gamma-ray interaction in the silicon layers is very low, resulting in low detection efficiency. A Compton camera might be more useful as part of active interrogation system, especially with an extremely low-dose source.
II-C. Active Interrogation of HEU
Clandestine transport of nuclear materials is an enormous challenge to current detection methods. Passive sensing can be rendered largely ineffective through the use of shielding, which easily blocks the low-energy gammas emitted from highly enriched uranium (HEU), for example. To make things worse, HEU emits almost no neutrons. The solution is active interrogation, defined as bombardment with neutrons or gammas to induce fissions that in turn induce nuclear materials to self identify via fission signatures. Utilization of the prompt-induced emissions from an active interrogation measurement for detection of SNM is essential to any high-throughput screening strategy for inspection of items and containers entering the United States. A screening technique that combines neutron radiography with measurement of prompt- and delayed-induced emissions can reduce the frequency of false alarms toward zero. With the added capabilities of active-interrogation measurement come additional operational risks, such as increased occupational dose to operators and increased radiation exposure to cargo. Current research efforts have focused on high-dose, fixed-facility systems, which, even if successful in detection, will have limited application due to the inherent complexities and safety issues associated with accelerator operations.
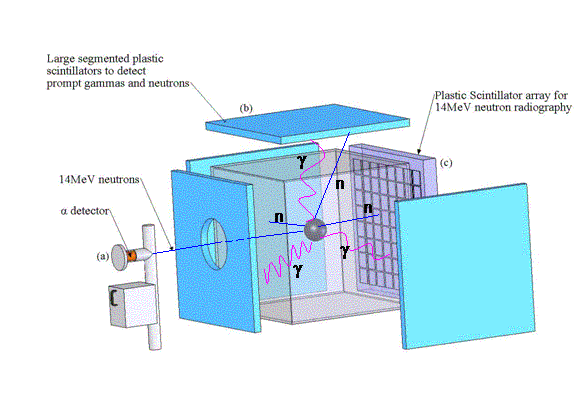
Figure 2. A schematic illustration of a field measurement using TANIS.11 The neutron generator (a) creates 14-MeV neutrons with a precisely known start time and direction. Plastic scintillator detector modules (b) are arrayed about the sample as geometry permits to detect induced-fission neutrons and gamma rays. A 2-dimensional plastic scintillator array (c) detects 14-MeV transmitted neutrons for neutron radiography. Delayed emissions are measured immediately after the neutron generator is turned off.
Another effort seeks to develop a portable, low-dose, tagged-active-neutron interrogation system (TANIS) for the detection and characterization of SNM such as HEU in cargo.11 The key to this approach is using time-of-flight and directional coincidence gating on alpha-tagged 14-MeV neutrons from the d + t → 4He + n reaction to improve the signal-to-noise ratio in transmission and induced fission measurements. TANIS uses three distinct measurement methods: prompt emission measurements of neutrons and gammas, 14-MeV stereoscopic neutron radiography imaging, and delayed emissions. As shown schematically in Figure 2, a cargo container is scanned in a plastic scintillator neutron and gamma-ray detector array, surrounded by a tagged-neutron (d,t) generator. Small items can be inspected all at once, while larger containers can be inspected in stages.
II-D. Other improvements
Improved analysis algorithms using Material Basis Set (MBS): There are already thousands of hand-held detectors used by first responders for radiation monitoring. Many commercially available radioisotope identifiers perform poorly. Tests by Blackadar et al. revealed that these radioisotope identifiers worked only 38% of the time.12 Performance of these hand-held units improved drastically when we introduced much-improved algorithms. One such algorithm, Material Basis Set (MBS), compensates for shielding impacts on gamma spectra, providing more accurate isotope identification.13 Running these algorithms does not impact detector performance in that typical calculation take only one second on the Motorola 64K series. When MBS was added to CdZnTe- and NaI based radioisotope identifiers, false alarms dropped to ~8%.
Many activities do not involve new detection principles. Instead, performance enhancements due to digital techniques and computational power result in more useful detectors and detector systems. For example, LANL is working on a portable neutron spectrometer in which a liquid scintillator packaged with digital electronics is placed into a hand-held portable unit with low power demands. Pulse-shape discrimination is applied to separate the contributions of neutrons from those of gamma rays. Both experimental calibrations and computational modeling have been used to derive response functions over a wide range of neutron energies that are needed in the deconvolution of fast-neutron spectra. A feasible next step would extend the instrument to the accurate measurement of radiation dose from fast neutrons. By incorporating tables of fluence-to-dose factors for neutrons as a function of their energy, on-board calculations from the measured energy spectrum could give accurate measurements of neutron dose in varying neutron fields that are difficult to obtain using other means.14
III. Summary
Homeland security requires low-cost, large-area detectors that can locate and identify weapons-usable nuclear materials and monitors for radiological isotopes that are more robust than current systems. Potential improvements can result from recent advances in electronics materials and nanotechnology, specifically nano-particle composite materials, organic semiconductors and inorganic quantum dots. This overview presented some recent initiatives in radiation detection using these new materials in the design of new device structures. Detector improvements demand not only new materials but also enhanced data-analysis tools that reduce false alarms, thereby increasing the quality of decisions. Additional computing power on hand-held platforms should enable the application of advanced algorithms to radiation detection in the field, reducing the need to transmit data and thus delay analysis. The need is for detectors that are bigger and more robust, not to mention cheaper, yet approach the theoretical efficiency and resolution limits of the component materials more closely than existing materials.
References
1. U. S. Customs and Border Protection: http://www.cbp.gov/xp/enforcement/international_activities/csi
2. http://abcnews.go.com/sections/wnt/DailyNews/sept11_uranium020911.html
3. http://abcnews.go.com/sections/wnt/PrimeTime/sept11_uranium030910.html
4. Liz Sidoti, ABC News, http://abcnews.go.com/Politics/wireStory?id=1774306
5. Ed McKigney et al., “Composite Scintillators for Radiation Detection and Nuclear Spectroscopy”, to be presented at SORMA XI and published in the proceedings (2006)
6. Ian H. Campbell and Brian K. Crone, “Quantum dot/organic semiconductor composites for radiation detection”, Advanced Materials, Vol. 18, Issue 1, pp. 77-79a
7. J.N. Ullom et al., “Development of Large Arrays of Microcalorimeters for Precision Gamma-ray Spectroscopy”, IEEE-NSS conference, Puerto Rico, October, 2005
8. Bill Murray et al., Patent US6781134
http://www.lanl.gov/news/releases/archive/03-150.shtml
http://www.lanl.gov/opportunities/techtransfer/dsp_technology.php?id=162
9. Gary Gardner et al., “Miniature Self-contained CdZnTe Gamma Spectrometer for use in Unattended Nuclear Material Monitoring”, IEEE-NSS conference, Puerto Rico, October, 2005
10. Gary Gardner, private communication, March (2006)
11. Bill Myers et al., “A Tagged Active Neutron Interrogation System (TANIS) For the Detection of Fissile Material in Cargo”, 2007106DR, Directed Research proposal for the Laboratory Directed Research Development effort (2006)
12. John Blackadar et al., RadAssessor, LA-CC-04-126 (2006)
13. Robert Estep et al., "Methods For Generating MBS (Material Basis Set) Basis Spectra For Use In Mass-Produced Handheld Monitor Systems," Proc. INMM 46th Annual Meeting, July 10-14, 2005, Phoenix, AZ
14. Mark Nelson, LANL, private communication, March (2006)